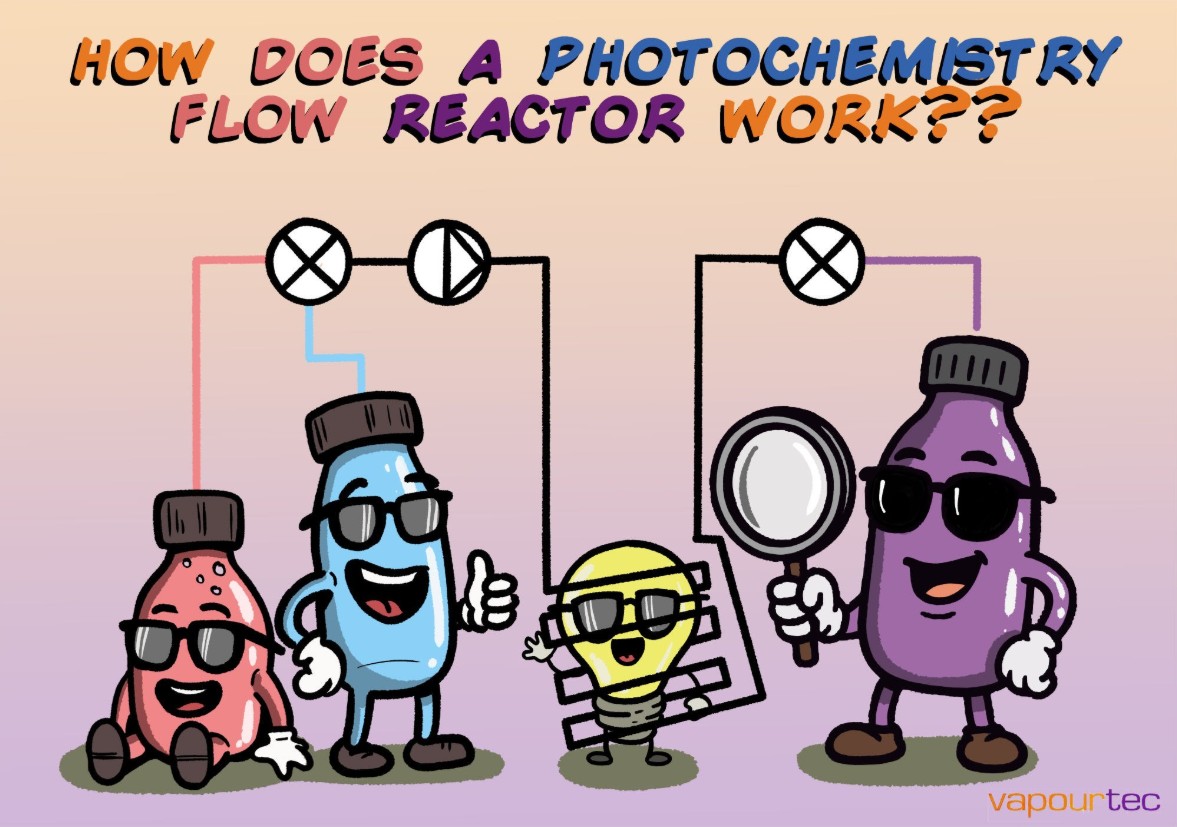
Date: 1 April 2025
Photochemistry flow reactors deliver light directly into liquid-phase reactions with unmatched precision. These systems drive chemical transformations continuously, using focused light and controlled flow to boost efficiency, safety, and scalability. This article explains their design and function – step by step- to show exactly how they operate and why they matter in modern synthetic chemistry.
What Is a Photochemistry Flow Reactor?
A photochemistry flow reactor channels liquid-phase reactants through narrow, often micro-structured conduits exposed to controlled light sources, typically LEDs or lasers tuned to specific wavelengths. This geometry maximises photon flux per unit volume and ensures high surface-area-to-volume ratios, which improves photon absorption efficiency compared to batch systems. By maintaining laminar flow and tightly regulating residence time, these reactors achieve consistent irradiation across the reaction medium. This uniform exposure eliminates gradient-driven inefficiencies—like localized overheating or incomplete conversion—that limit batch setups. Flow platforms also allow precise modulation of reaction parameters such as temperature, pressure, and flow rate, which makes them indispensable for scaling photochemical processes while preserving selectivity and reproducibility.
Key Components and How They Work
1. Light Source
The heart of any photoreactor is its light source, usually either a UV lamp (low- or medium-pressure mercury) to provide broad-spectrum UV light or LED arrays to deliver wavelength-specific light efficiently, often from 365 to 450 nm. In reactors like the Vapourtec UV-150, these light sources are housed in interchangeable modules, allowing chemists to tune the wavelength to match their reaction requirements.
2. Reactor Path
This is the actual route that the reactants travel through. It may comprise a coiled FEP or quartz tubing around the lamp (e.g., Vapourtec’s setup), or microfluidic chips illuminated from above/below by LEDs
The reactor path is made from UV-transparent materials so photons can penetrate and trigger chemical reactions.
3. Housing and Optical System
This outer enclosure:
- Shields users from harmful UV radiation
- Often includes reflective surfaces or dichroic mirrors, which reflect UV light back into the reactor while allowing heat (IR) to escape
- Improves efficiency by recycling stray photons and minimizing energy loss
The UV-150, for example, reflects over 90% of UV light back into the reaction zone while venting IR heat, optimizing both reaction performance and thermal safety.
4. Temperature Control
Photochemical reactions can generate significant heat. Many reactors include:
- Air cooling systems (fans or ducts)
- Water jackets or thermal blocks
This ensures that heat from high-energy lamps (which can reach 600 °C) doesn’t degrade reactants or skew reaction conditions. Temperature stability is critical for reproducible photochemistry.
5. Pump and Flow Control
While technically external, flow control is vital. Pumps deliver reactants through the reactor at a defined flow rate, which determines residence time (how long the solution is irradiated). Back-pressure regulators may also be used to maintain pressure and prevent gas or vapour bubbles forming during the reaction.
Operating Principle: Step-by-Step
- Loading the System: A reservoir is filled with reactant solution and connected to a pump.
- Initiating Flow: The pump drives the solution through the reactor’s flow path.
- Illumination and Reaction: As the solution travels through the transparent tubing or channels, it’s exposed to the light source. Molecules absorb photons and enter excited states, initiating the desired photochemical transformations.
- Temperature Stabilization: Active cooling keeps the reactor at the desired setpoint (e.g., 25 °C), ensuring that heat from the light doesn’t disrupt the reaction.
- Product Collection: The reacted solution exits the reactor and is collected or sent to downstream processing. Because the flow is continuous, the reactor can run for hours, producing scalable quantities of product.
Reactor Design Variations
- Coil Reactor with Lamp: Like the UV-150, where a coiled tube wraps around a central lamp. This ensures uniform irradiation and scalable residence time based on coil length.
- Microreactor with LEDs: Compact, chip-based design for small-scale or high-throughput experiments. LEDs provide direct, even illumination.
Batch vs. Flow Operation
Feature | Batch Photochemistry | Flow Photochemistry |
---|---|---|
Light exposure | Uneven across large volumes | Uniform across narrow channels |
Scale-up | Complex and often inefficient | Simple: run longer or in parallel |
Temperature control | Challenging over time | Active cooling in real time |
Reproducibility | Variable due to mixing, exposure | High due to consistent residence time |
Practical Example Workflow
Let’s say you’re performing a 365 nm photochemical oxidation:
- Step 1: Prepare your solution (substrate, catalyst, solvent).
- Step 2: Set the pump to 0.5 mL/min.
- Step 3: Select a 365 nm LED module on the UV-150.
- Step 4: Maintain reactor at 25 °C using forced-air cooling.
- Step 5: As the solution flows through the coil (5-minute residence time), it turns blue, signalling product formation. The outflow is collected in a flask.
Because the conditions are fixed, each portion of fluid sees exactly 5 minutes of light – resulting in consistent conversion and minimal by-products.
Adjusting the Conditions
- Light intensity: Adjust via power settings or select higher-output bulbs.
- Wavelength: Swap LED or mercury lamp modules.
- Flow rate: Slower flow = longer residence time = more conversion.
- Temperature: Controlled through built-in cooling/heating.
Final Thoughts
Photochemistry flow reactors, such as the Vapourtec UV-150, are designed with precision engineering to deliver reliable, efficient, and safe light-driven synthesis. Their modularity, control systems, and built-in safety features make them indispensable tools in the modern lab.
By understanding how these components work together—from light delivery to temperature control – you can run your photochemical reactions with greater confidence and scalability. Want to see a photochemistry flow reactor in action? Contact us if you would like to learn more.