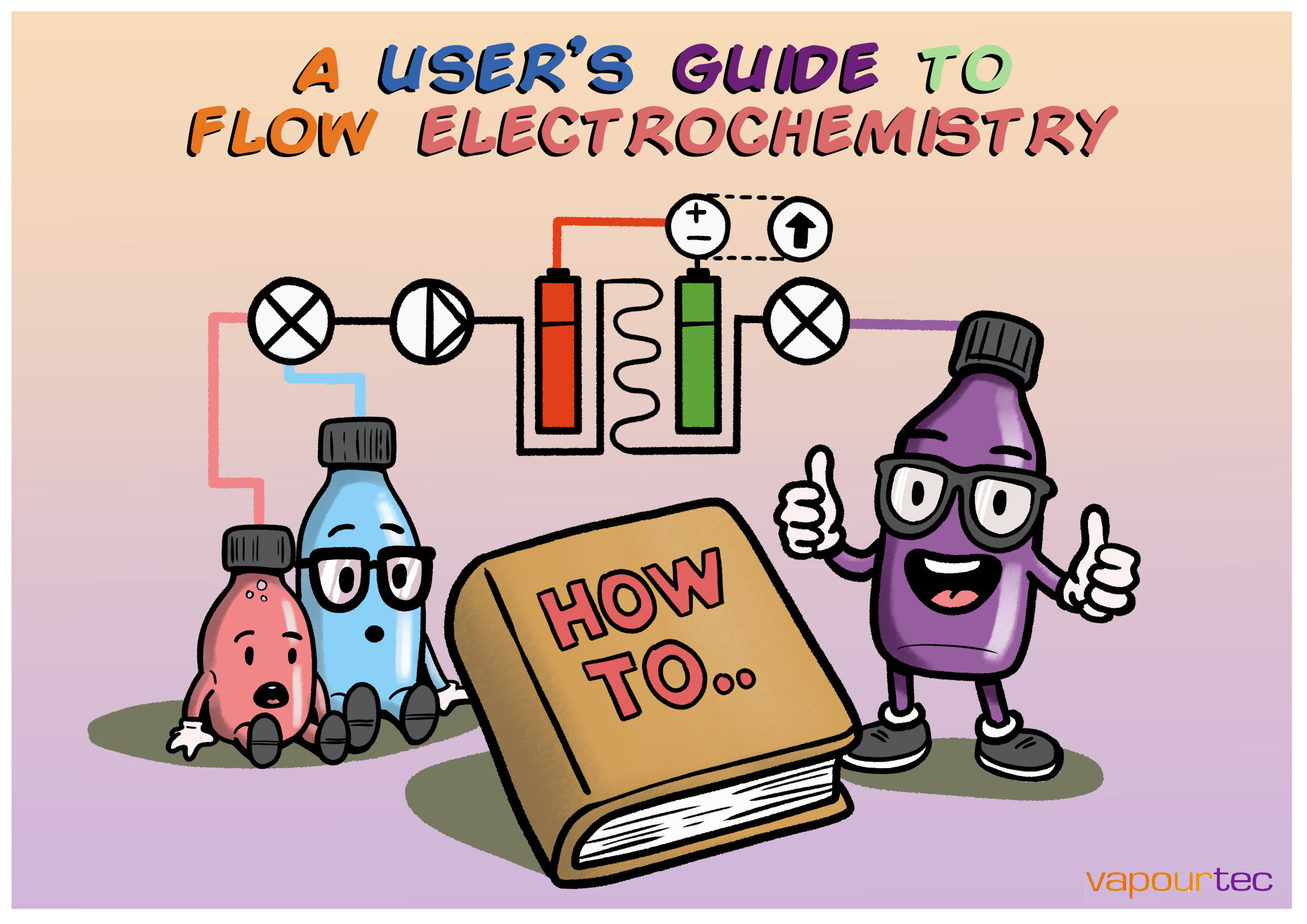
Date: 1 January 2025
Plug electrons into a continuous flow, and chemistry starts to move faster—literally. Flow electrochemistry fuses precise redox control with the streamlined design of continuous processing. This setup dramatically reduces reaction times, sharpens selectivity, and simplifies scale-up without compromising safety. Engineers and chemists now shape molecules more cleanly, manage heat and mass transfer more efficiently, and design safer systems for both small-batch labs and industrial reactors. Whether building complex organic structures or breaking down pollutants, this method gives researchers a more powerful and flexible way to drive chemical transformations. But how?
Whether you’re new to flow electrochemistry or an experienced practitioner seeking advanced insights, this guide will equip you with the fundamental principles, practical techniques, best practices, and troubleshooting strategies needed to effectively design and execute electrochemical reactions in flow.
Why Choose Flow Electrochemistry?
Electrochemistry uses electricity to drive redox reactions, substituting hazardous oxidants or reductants with clean, controllable electrons. In continuous flow, reagents are pumped through a reactor that houses electrodes. This setup eliminates the limitations of batch processes, such as poor mass transfer or thermal control, by enabling consistent exposure to an electric field and efficient heat dissipation.
Flow electrochemistry isn’t just safer—it’s more reproducible, scalable, and energy-efficient. For chemists aiming to minimize waste, improve yields, or handle reactive intermediates, flow-based electrochemical systems are quickly becoming indispensable.
Flow electrochemistry is powering advances in:
- Oxidations and reductions of organic molecules
- Fluorinations, often difficult or hazardous in batch
- Sustainable synthesis using renewable electricity
For example, our systems have enabled safe, scalable fluorination reactions with better selectivity and reduced environmental footprint compared to batch methods.
From Batch to Flow: The Fundamentals
Traditional batch electrolysis involves immersing electrodes in a beaker of electrolyte. It works, but scale-up is tough, and reaction conditions are hard to maintain. In contrast, flow electrochemistry uses a compact, modular reactor where reagents pass between parallel plate electrodes.
These flow cells ensure:
- Consistent inter-electrode distance (for controlled current density)
- Small volumes for enhanced safety
- High surface-area-to-volume ratio (better mass transfer and heat exchange)
Electrons, acting as reagents, transfer at the anode and cathode, driving oxidation and reduction reactions. This precision, coupled with automation, allows for tighter reaction control.
What You Need to Get Started
A typical setup includes:
- Pump systems: To deliver reagents at controlled flow rates
- Electrochemical reactor: Often parallel plate design; can be divided (with membrane) or undivided
- Electrodes: Materials like carbon, platinum, nickel, or boron-doped diamond
- Power supply: Galvanostat (constant current) or potentiostat (constant voltage)
- Supporting electrolyte: Ensures conductivity without interfering with the reaction
Operating Parameters: What to Control
To optimize a reaction, pay close attention to:
- Electrode material: Impacts selectivity and efficiency
- Flow rate: Determines residence time in the cell
- Current or voltage: Sets the reaction rate and selectivity
- Temperature and pressure: Affects solubility and kinetics
- Cell configuration: Divided cells prevent product recombination; undivided cells are simpler
Deciding between galvanostatic (constant current) and potentiostatic (constant voltage) modes depends on your reaction goals. Galvanostatic operation often simplifies scale-up, while potentiostatic control can help prevent side reactions.
Step-by-Step: Running Your First Experiment
Prepare the system:
- Clean electrodes
- Load reagents
- Prime flow lines
Set conditions:
- Select current or voltage
- Choose flow rate
- Adjust temperature and pressure (if needed)
Start the reaction:
- Monitor cell voltage (in constant current mode) or current (in constant voltage mode)
Track conversion with inline or offline analysis
Optimize:
- If conversion is low, reduce flow rate or increase applied potential
- If side products appear, change electrode material or tweak electrolyte
Troubleshooting and Best Practices
Here are just a few common issues users often encounter with flow electrochemistry with some simple troubleshooting tips that could help. Please remember that the exact nature of your experiment could alter these best practices.
Low conversion?
- Lower flow rate to increase residence time
- Increase electrode surface area
Electrode fouling?
- Try different materials
- Use periodic polarity reversal or chemical cleaning
Gas evolution issues?
- Use a back-pressure regulator
- Vent gases safely
Short-circuits or overheating?
- Ensure no metallic contacts are exposed
- Monitor temperature closely
Thinking Ahead: What’s Next?
Flow electrochemistry doesn’t just improve on batch—it breaks new ground. It handles what batch can’t: tight integration with photochemistry, precise gas-liquid control, and streamlined multi-step syntheses. With systems like the Vapourtec Ion reactor, built to slot into both E-Series and R-Series platforms, labs gain full automation, real-time data, and plug-and-play electrochemistry at scale.
If you’re exploring your first electrosynthetic step or building toward production, flow technology clears the path. It cuts waste, sharpens control, and brings high-end electrochemistry into everyday use. Let us help you make the transition seamless.